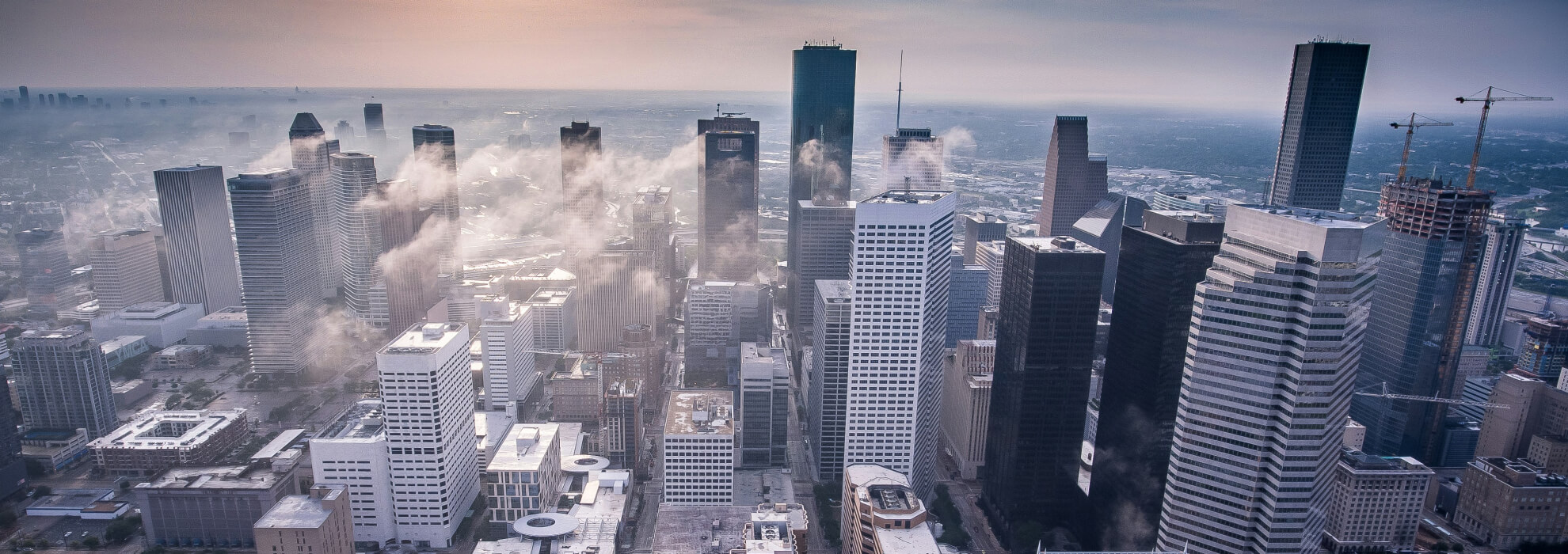
Watch short for this article (5 slides)
Celestial Dance: Unveiling the Science and Wonder of the Aurora Borealis
The night sky near Earth's poles occasionally transforms into a breathtaking canvas painted with ethereal, shifting curtains of light – the Aurora Borealis (Northern Lights) and its southern counterpart, the Aurora Australis. For millennia, these mesmerizing displays have captivated observers, inspiring myths of celestial spirits, divine battles, and spectral dancers. While modern science has demystified the aurora, explaining it as a stunning interplay between the Sun and Earth's atmosphere, the sense of wonder remains. What exactly triggers this celestial light show, why does it favor the polar regions, and what creates its vibrant palette of colors?
Photo by Jonatan Pie on Unsplash - The aurora illuminates the polar night sky.
The Sun's Influence: The Solar Wind
The aurora's journey begins nearly 150 million kilometers (93 million miles) away, at our Sun. The Sun constantly emits a stream of charged particles – primarily electrons and protons, with some heavier ions – known as the solar wind. This plasma travels outward through the solar system at speeds typically ranging from 400 to 800 kilometers per second (about 1 to 2 million miles per hour).
The intensity and speed of the solar wind are not constant. They fluctuate based on solar activity, including the Sun's ~11-year cycle. During periods of high activity (solar maximum), events like solar flares (intense bursts of radiation) and especially Coronal Mass Ejections (CMEs) (massive eruptions of plasma and magnetic fields from the Sun's corona) can significantly enhance the density and speed of the solar wind particles hurtling towards Earth.
Earth's Magnetic Shield: The Magnetosphere
As the solar wind approaches Earth, it first encounters our planet's magnetic field, the magnetosphere. Generated by the motion of molten iron in Earth's outer core (a dynamo effect), the magnetosphere acts like a protective bubble, deflecting the vast majority of the incoming charged solar wind particles around our planet. The magnetosphere is compressed on the dayside (facing the Sun) and stretched into a long tail (the magnetotail) on the nightside.
However, this shield isn't perfect. The magnetosphere has vulnerabilities, particularly near the magnetic poles. Earth's magnetic field lines curve downwards into the atmosphere in funnel-like regions known as the polar cusps. Charged particles from the solar wind can become trapped within the magnetosphere, particularly in the magnetotail, and then accelerated along these magnetic field lines towards the polar regions.
Earth's magnetosphere deflects most solar wind but funnels some particles toward the poles along magnetic field lines, creating the auroral ovals.
Atmospheric Collisions: The Light Show Begins
The visible aurora occurs when these high-energy electrons (and to a lesser extent, protons) channeled down the magnetic field lines collide with gas molecules and atoms in Earth's upper atmosphere, primarily molecular nitrogen (Nâ‚‚) and atomic oxygen (O). These collisions typically occur at altitudes ranging from about 80 to over 600 kilometers (50 to 400 miles).
The process unfolds through basic atomic physics:
- Excitation: The incoming high-energy particle transfers energy to the atmospheric gas atom or molecule upon collision. This energy kicks an electron within the atom/molecule into a higher, unstable energy level or state.
- Emission: To return to its stable, lower-energy ground state, the excited atom/molecule must release this excess energy. It does so by emitting a tiny packet of light – a photon.
The collective light emitted by billions upon billions of these individual atomic and molecular collisions creates the aurora we see.
The Palette of the Poles: Explaining Auroral Colors
The specific colors observed in an aurora depend crucially on two factors: the type of gas molecule being excited and the altitude at which the collision occurs (which influences the energy state the gas particle is excited to and how long it stays excited before emitting light).
- Green (Most Common): Produced by collisions with atomic oxygen (O) at altitudes typically between 100 and 300 kilometers (60 to 185 miles). This specific green wavelength (557.7 nanometers) is very common because atomic oxygen is abundant at these altitudes, and the excited state responsible has a relatively short lifetime (about 0.7 seconds), meaning it emits light fairly quickly.
- Red: Also produced by atomic oxygen (O), but at much higher altitudes (typically above 300 kilometers / 185 miles). At these heights, the atmosphere is thinner, and collisions are less frequent. Oxygen atoms excited to a different, higher energy state can remain there for a longer time (up to two minutes) before emitting a photon at red wavelengths (630.0 and 636.4 nanometers). This color often appears at the upper fringes of strong auroral displays.
- Blue and Purple/Violet: Generally produced by collisions with molecular nitrogen (Nâ‚‚). Excited nitrogen molecules tend to emit light almost instantaneously. Blues (around 427.8 nm) and purples/violets often appear at the lower edges of auroral curtains, sometimes below 100 kilometers (60 miles), especially during very energetic events where particles penetrate deeper into the atmosphere. Nitrogen can also emit reddish light at higher altitudes under certain conditions.
- Pink: Often a mix of green and red light, or sometimes related to specific nitrogen emissions, appearing at lower altitudes.
(Source: NOAA Space Weather Prediction Center - Aurora; Source: NASA - Aurora Poster/Explanation)
Where and Why: The Auroral Ovals
The aurora doesn't occur uniformly over the poles. The regions where energized particles precipitate into the atmosphere form dynamic, ring-shaped zones known as the Auroral Ovals, centered around Earth's geomagnetic poles (which are offset slightly from the geographic poles).
The size and location of these ovals are constantly changing, driven by the strength and configuration of the solar wind and Earth's magnetosphere. During periods of low geomagnetic activity, the ovals are smaller and confined to higher latitudes. During strong geomagnetic storms (triggered by CMEs or high-speed solar wind streams), the ovals expand significantly equatorward, allowing auroras to be seen at much lower latitudes than usual.
- Prime Northern Hemisphere Locations (within or near the typical oval): Alaska (USA), Northern Canada (Yukon, Northwest Territories, Nunavut), Greenland, Iceland, Northern Norway, Sweden, Finland, Northern Siberia.
- Prime Southern Hemisphere Locations: Antarctica, Tasmania (Australia), South Island of New Zealand, southern tips of Argentina and Chile (less commonly observed due to more ocean coverage).
Timing is Key: When to Witness the Aurora
- Darkness is Essential: Auroras occur year-round, day and night, but they are only visible to the naked eye against a dark night sky. This is why the long, dark nights of winter in polar regions (roughly September to April in the north, March to September in the south) offer the best viewing opportunities.
- Solar Activity Matters: While auroras can occur even during quiet solar periods, they are generally more frequent, intense, and geographically widespread during periods of higher solar activity, particularly around the peak of the ~11-year solar cycle and following significant solar events like CMEs.
- Geomagnetic Forecasts: Space weather agencies provide short-term aurora forecasts, often using the Planetary K-index (Kp), a measure of global geomagnetic activity ranging from 0 (calm) to 9 (extreme storm). Higher Kp values (typically Kp 4 or 5 and above) indicate stronger geomagnetic disturbances and a higher likelihood of visible aurora at lower latitudes.
- Time of Night: While auroras can appear anytime during darkness, activity often peaks around local magnetic midnight (which differs slightly from local midnight based on your position relative to the geomagnetic pole).
Myths and Legends: Cultural Interpretations
Before scientific understanding, cultures interpreted the aurora through their own cosmologies:
- Norse Mythology: Some interpretations link the aurora to the Bifröst bridge connecting Earth and Asgard, or to the light reflecting off the shields of the Valkyries carrying fallen warriors to Valhalla.
- Inuit Cultures: Various beliefs existed, including the idea that the lights were the spirits of the dead playing a game with a walrus skull, or torches held by spirits guiding newcomers to the afterlife.
- Sami People (Northern Scandinavia): Traditionally viewed the aurora with respect and sometimes fear, believing the lights were ancestral spirits and that disrespecting them (e.g., whistling or waving) could bring misfortune.
- Finnish Folklore: The name "revontulet" translates to "fox fires," stemming from a myth about an arctic fox running across the fells, its tail sweeping sparks into the sky.
Tips for Aurora Hunters
- Go North (or South): Travel to locations within or near the typical auroral ovals.
- Embrace Darkness: Seek locations far from city light pollution. New moon phases are ideal.
- Check the Forecast: Monitor space weather forecasts (Kp index and specific aurora forecasts).
- Be Patient: Auroral displays are unpredictable; activity can wax and wane throughout the night. Dress warmly and be prepared to wait.
- Look North (Usually): In the Northern Hemisphere, the aurora often begins as a glow or arc on the northern horizon, potentially moving higher or overhead if activity increases.
Conclusion: Nature's Grand Light Show
The Aurora Borealis and Australis are stunning demonstrations of the intricate connection between the Sun, Earth's magnetic field, and our atmosphere. Driven by the solar wind and guided by our planet's magnetosphere, collisions between energetic particles and atmospheric gases paint the polar skies with vibrant, dancing light. While science now explains the mechanisms – from magnetic reconnection to atomic excitation and photon emission – the sheer beauty and dynamic nature of the aurora continue to inspire awe and wonder, bridging the gap between ancient myth and modern understanding of our place in the cosmos.