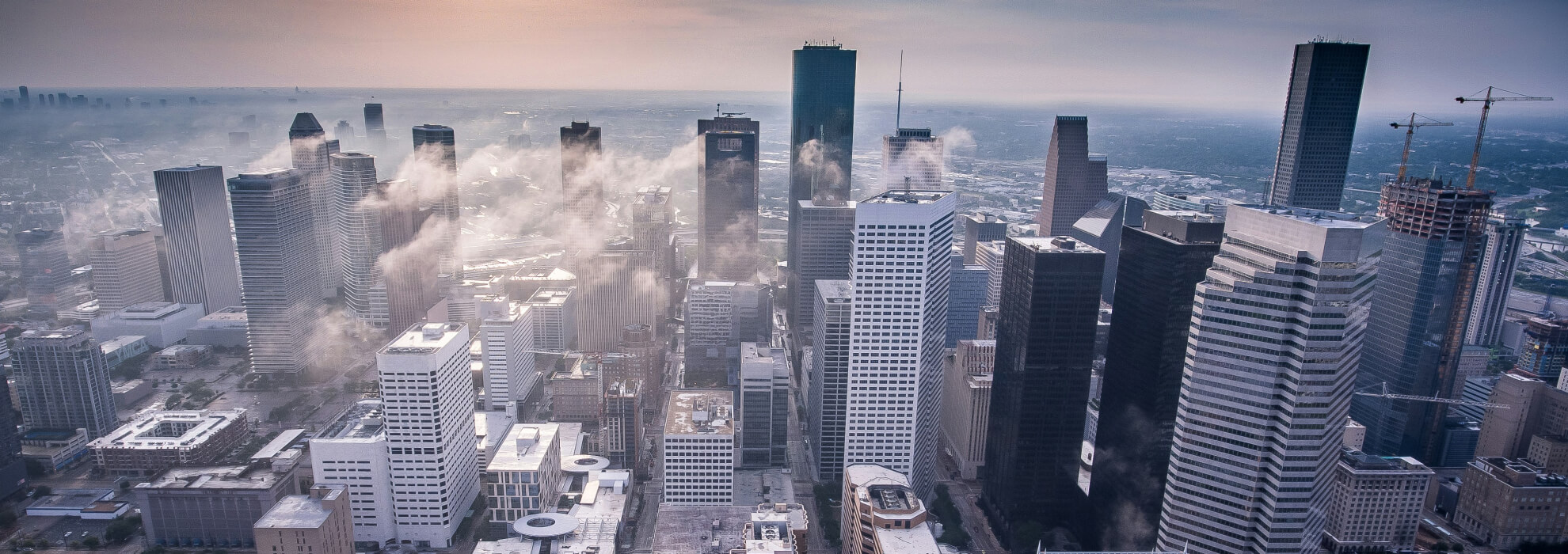
Watch short for this article (5 slides)
Cosmic Encounters: Could Interstellar Clouds Reshape Earth's Atmosphere?
Our Solar System's journey through the Milky Way galaxy is not a solitary voyage through empty space. It periodically traverses vast, diffuse regions filled with gas and dust known as interstellar clouds. While much scientific attention has focused on the potential link between these encounters and past climate changes, particularly ice ages, the influence of these cosmic clouds might extend deeper, potentially altering the composition, chemistry, and protective layers of Earth's atmosphere itself. Understanding these interactions offers a fascinating glimpse into how events far beyond our planet could shape the conditions necessary for life.
What are Interstellar Clouds? Drifting Giants of Gas and Dust
Interstellar clouds are enormous accumulations of gas (primarily hydrogen and helium, with trace amounts of other elements) and microscopic dust particles found in the space between stars within a galaxy. They vary dramatically in size, density, temperature, and composition:
- Diffuse Clouds: Relatively low density and warmer (thousands of degrees Kelvin), often composed mainly of neutral or ionized hydrogen.
- Molecular Clouds: Denser and much colder (10-30 Kelvin), allowing molecules (like H₂, CO, and more complex organic molecules) to form. These are the birthplaces of stars.
- Composition: Primarily gas (~99%) and dust (~1%). The dust consists of tiny solid grains (silicates, carbon compounds, ice mantles).
The Solar System, in its ~230 million-year orbit around the galactic center, inevitably passes through various types of these clouds. The density of the local interstellar medium (LISM) surrounding our Solar System varies, and encounters with denser clouds are statistically expected over geological timescales.
The Heliosphere: Our Solar System's Protective Bubble
To understand how interstellar clouds interact with Earth, we must first consider the **heliosphere**. This is a vast magnetic bubble created by the solar wind – a continuous stream of charged particles flowing outward from the Sun. The heliosphere shields the Solar System, including Earth, from much of the harsh radiation environment of interstellar space, particularly lower-energy **galactic cosmic rays (GCRs)** – high-energy particles originating from supernovae and other energetic events outside the Solar System.
The size and shape of the heliosphere are determined by the balance between the outward pressure of the solar wind and the inward pressure of the surrounding interstellar medium. Encounters with dense interstellar clouds can compress the heliosphere, potentially pushing its boundary (the heliopause) closer to the inner Solar System, including Earth's orbit.
Potential Atmospheric Impacts of Interstellar Cloud Encounters
When the Solar System moves through a sufficiently dense interstellar cloud, several potential effects on Earth's atmosphere are hypothesized:
1. Altered Cosmic Ray Exposure and Atmospheric Chemistry
This is perhaps the most studied potential link.
- Compression of the Heliosphere: A dense cloud pushing against the heliosphere could weaken its shielding effect, allowing more GCRs to penetrate the inner Solar System and reach Earth's atmosphere.
- Direct Cloud Influence: Some theories suggest dense clouds themselves might contain embedded magnetic fields that could funnel or even generate additional cosmic rays, although this is more speculative. Conversely, very dense clouds might offer some temporary *shielding* from external GCRs if the Solar System were fully enveloped.
- Atmospheric Consequences of Increased GCRs:
- Cloud Formation: GCRs ionize atmospheric molecules. Some hypotheses (like Svensmark's 'cosmoclimatology') propose that this ionization aids the formation of cloud condensation nuclei (CCN), potentially leading to increased low-level cloud cover. Increased cloud cover could have a net cooling effect (reflecting sunlight) or warming effect (trapping heat), depending on cloud type and altitude, thus influencing climate. This link remains highly debated within the climate science community. (Space.com article discussing cosmic ray/climate link).
- Atmospheric Chemistry Changes: Increased ionization by GCRs can affect atmospheric chemistry, potentially altering concentrations of trace gases and contributing to the formation of aerosols.
2. Ozone Layer Disruption
Earth's ozone layer (primarily in the stratosphere) protects life by absorbing harmful ultraviolet (UV) radiation from the Sun.
- Mechanism: Extremely high influxes of GCRs, or potentially associated energetic particles trapped in the compressed heliosphere or within the cloud itself, could produce secondary particles (like neutrons and muons) upon hitting the upper atmosphere. These secondary particles, along with the direct ionization effects, can catalyze chemical reactions that deplete stratospheric ozone (similar in mechanism, though different in source particle, to ozone depletion caused by solar proton events).
- Potential Consequences: Significant ozone depletion would allow more harmful UV-B radiation to reach the surface, increasing risks of skin cancer, cataracts, and damage to ecosystems (particularly phytoplankton, the base of marine food webs). Some researchers have speculated whether past mass extinction events might correlate with periods of significantly weakened geomagnetic field (reducing Earth's own magnetic shielding) combined with potential increases in GCRs during interstellar cloud passages or nearby supernovae. (Example study linking extinctions and astrophysical events).
3. Changes in Atmospheric Composition (Accretion)
As Earth moves through a dense cloud, it could directly accrete interstellar gas and dust.
- Dust Influx: Increased influx of interstellar dust particles into the upper atmosphere could potentially affect atmospheric chemistry, act as CCN influencing cloud formation, or alter the atmosphere's radiative balance. Studies analyze sediment layers and ice cores for isotopes like Helium-3 (³He, rare on Earth but more common in space) or Iron-60 (⁶⁰Fe, produced in supernovae and found in interstellar dust) to search for evidence of past accretion events.
- Gas Accretion: While Earth's atmosphere is dense, some direct accretion of interstellar gas (particularly hydrogen) into the exosphere and upper atmosphere is possible, though likely insufficient to cause major compositional shifts unless the cloud is exceptionally dense and the encounter prolonged.
Evidence and Challenges: Piecing Together the Cosmic Puzzle
Finding definitive proof of these interactions is challenging:
- Paleoclimate and Geological Records: Scientists search for correlations between indicators of past interstellar cloud encounters (e.g., peaks in ³He or ⁶⁰Fe isotopes in deep-sea sediments or ice cores) and simultaneous changes in climate proxies (temperature records from ice cores, ocean sediments), biodiversity records (extinction events), or atmospheric composition indicators. Establishing causality is difficult, as other factors (volcanism, internal climate variability) are always at play.
- Modeling the Heliosphere and ISM: Computer models simulate the interaction between the solar wind, the heliosphere, and different types and densities of interstellar clouds to predict changes in GCR flux reaching Earth. These models rely on assumptions about cloud properties and the heliosphere's structure.
- Astronomical Observations: Studying the properties of the LISM and mapping nearby interstellar clouds helps estimate the likelihood and nature of past and future encounters. Spacecraft like Voyager 1 & 2, now in interstellar space, provide direct measurements of the environment outside the heliosphere.
The primary challenge lies in disentangling the potential subtle signal of an interstellar cloud encounter from the much larger signals of internal Earth system changes and other astronomical factors over geological time.
Future Research: Probing Our Cosmic Neighborhood
Further investigation is needed to clarify the role of interstellar clouds:
- Improved Isotopic Analysis: Higher-resolution analysis of geological and ice core records for cosmic dust tracers (⁶⁰Fe, Plutonium-244) and cosmogenic isotopes (like Beryllium-10, produced by GCR interactions in the atmosphere) can provide a more detailed timeline of past GCR fluctuations and dust influx events.
- Advanced Modeling: Incorporating more sophisticated MHD physics and realistic interstellar cloud properties into heliospheric models to better simulate GCR modulation and atmospheric interactions. Linking these models with climate and atmospheric chemistry models.
- Direct Interstellar Probes: Future dedicated interstellar probe missions could directly sample the composition and properties of the LISM and nearby clouds, providing crucial data to constrain models.
- Cosmic Ray Monitoring: Continued precise monitoring of GCR flux reaching Earth helps understand current variations and potentially identify influences from the local interstellar environment.
Conclusion: Earth's Atmospheric Connection to the Galaxy
While the effects of interstellar cloud encounters on Earth's climate remain an area of active research and debate, their potential to influence our atmosphere more broadly – affecting cosmic ray shielding, ozone chemistry, and even composition through dust accretion – presents a compelling field of study. These cosmic events highlight that Earth is not isolated but dynamically interacts with its galactic environment. Although the immediate impacts of climate change driven by human activity are far more pressing and certain, understanding how our planet responds to these long-term astrophysical influences provides valuable context for Earth's environmental history and its place within the wider cosmos. Further research bridging astrophysics, heliophysics, geology, and atmospheric science is needed to fully unravel the subtle but potentially significant ways interstellar clouds may have shaped the air we breathe.