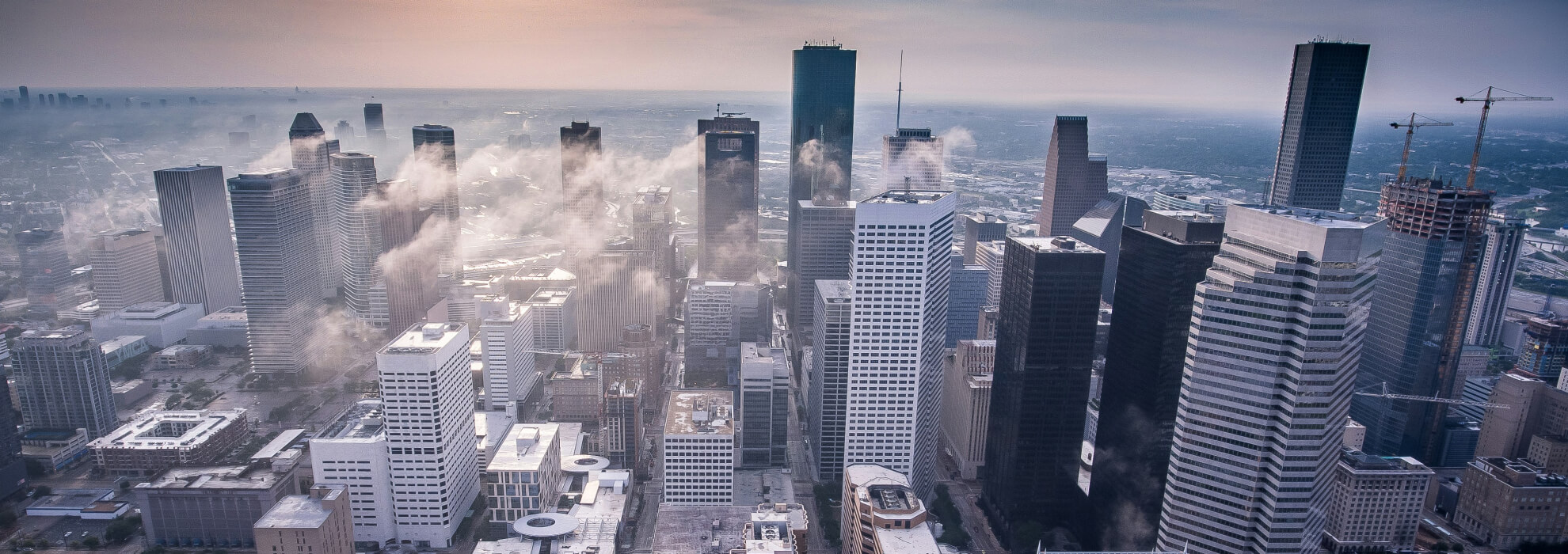
Watch short for this article (5 slides)
Living Libraries of the Sea: How Coral Reefs Archive Earth's Climate History
Beneath the shimmering surface of tropical oceans lie vibrant cities of life – coral reefs. These intricate ecosystems, teeming with biodiversity, are also invaluable natural archives, chronicling centuries of environmental change within their stony skeletons. While often discussed as fragile indicators under immediate threat from warming waters, their role extends far deeper. Corals act as high-resolution recorders, capturing subtle shifts in temperature, water chemistry, and weather patterns long before human instruments were widespread. By deciphering these skeletal records, scientists can reconstruct past climate variability, understand the context of current changes, and refine predictions for our planet's future under accelerating climate change.
Photo by QUI NGUYEN on Unsplash
The Coral Archivist: Building Skeletons, Recording Time
To understand how corals record climate, it's essential to grasp their basic biology:
- The Organism: Reef-building corals are colonies of tiny animals called polyps. Each polyp secretes a hard skeleton made primarily of calcium carbonate (CaCO₃), typically in the mineral form known as **aragonite**.
- Symbiosis: Most reef-building corals live in a vital symbiotic relationship with microscopic algae called zooxanthellae (dinoflagellates of the family Symbiodiniaceae) living within their tissues. These algae photosynthesize, providing the coral polyps with essential nutrients (sugars), while the coral provides shelter and compounds needed for photosynthesis. This symbiosis fuels rapid skeletal growth.
- Growth Patterns: As the colony grows, it deposits layers of aragonite, forming the reef structure. Like tree rings, these skeletons often exhibit annual density bands related to seasonal changes in growth rate (influenced by temperature, light, and potentially nutrient availability). These bands allow scientists to date different sections of the coral skeleton precisely.
It is within the chemical composition of these incrementally deposited skeletal layers that the climate record is encoded.
Decoding the Skeleton: Geochemical Proxies for Past Climates
Scientists analyze the chemical makeup of coral skeletons using various **geochemical proxies** – measurable properties that respond predictably to environmental variables. Key proxies include:
1. Strontium-to-Calcium Ratio (Sr/Ca): A Thermometer in the Skeleton
- Mechanism: As corals build their aragonite (CaCO₃) skeletons, trace amounts of strontium ions (Sr²⁺) substitute for calcium ions (Ca²⁺) in the crystal lattice. The degree of this substitution is temperature-dependent: **corals incorporate proportionally less strontium relative to calcium at higher water temperatures**.
- Application: By measuring the Sr/Ca ratio down a dated coral core using techniques like mass spectrometry, scientists can reconstruct past sea surface temperatures (SSTs) with remarkable accuracy (often within ±0.5°C or better). Long-lived, massive corals like *Porites* species can provide continuous SST records spanning several centuries. (Example NOAA Paleoclimatology Data from Coral Sr/Ca)
2. Oxygen Isotopes (δ¹⁸O): A Dual Signal of Temperature and Salinity
- Mechanism: Oxygen exists in different stable isotopes, primarily light oxygen-¹⁶ (¹⁶O) and heavier oxygen-¹⁸ (¹⁸O). The ratio of these isotopes (expressed as δ¹⁸O) in the coral's calcium carbonate skeleton is influenced by two main factors:
- Water Temperature: During carbonate precipitation, corals incorporate relatively more ¹⁸O at *colder* temperatures.
- Seawater δ¹⁸O: The isotopic composition of the surrounding seawater itself varies. Evaporation preferentially removes lighter ¹⁶O, leaving surface waters enriched in ¹⁸O (higher salinity often correlates with higher δ¹⁸O). Conversely, rainfall and freshwater runoff are depleted in ¹⁸O, lowering seawater δ¹⁸O (lower salinity often correlates with lower δ¹⁸O).
- Application: Coral δ¹⁸O provides a combined signal reflecting both temperature and the local hydrological cycle (salinity, rainfall/runoff). By comparing δ¹⁸O records with independent temperature reconstructions (like Sr/Ca), scientists can potentially disentangle these signals to infer past changes in rainfall patterns, river discharge, or large-scale ocean salinity shifts associated with phenomena like El Niño-Southern Oscillation (ENSO).
3. Boron Isotopes (δ¹¹B): Tracking Ocean Acidification
- Mechanism: Boron exists in seawater primarily as boric acid [B(OH)₃] and borate ion [B(OH)₄]⁻. The relative abundance of these two forms is pH-dependent. Corals incorporate boron (primarily the borate ion) into their skeletons, and the isotopic ratio of boron (¹¹B vs ¹⁰B, expressed as δ¹¹B) incorporated is related to the pH of the seawater at the time of calcification.
- Application: Analyzing δ¹¹B in dated coral cores allows scientists to reconstruct past ocean pH levels, providing direct evidence of long-term ocean acidification driven by the oceanic uptake of atmospheric CO₂. (MIT News Article on Coral Boron Isotopes)
4. Other Trace Elements (Ba/Ca, U/Ca, Mg/Ca, etc.)
- Barium/Calcium (Ba/Ca): Can serve as a proxy for nutrient upwelling or riverine runoff, as barium concentrations are often higher in deep water or river water compared to surface ocean water.
- Uranium/Calcium (U/Ca): Can potentially track changes in carbonate ion concentration or temperature, although the relationship is complex.
- Magnesium/Calcium (Mg/Ca): Also explored as a potential temperature proxy, though often more complex to interpret than Sr/Ca due to biological influences.
Insights from the Archives: What Corals Tell Us About Climate Change
Photo by Tom Fisk
Analysis of coral records from around the world has provided invaluable insights into past and present climate dynamics:
- Unprecedented Recent Warming: Coral Sr/Ca records consistently show that the rate and magnitude of ocean warming in the late 20th and early 21st centuries are unprecedented over the past several hundred years, confirming instrumental observations and extending them further back in time.
- Historical ENSO Variability: Coral δ¹⁸O records from the tropical Pacific have been instrumental in reconstructing the frequency and intensity of past El Niño and La Niña events, revealing natural cycles and helping scientists assess whether human activity is altering ENSO behavior.
- Documenting Ocean Acidification: Coral δ¹¹B records provide direct, quantitative evidence of the progressive decline in ocean pH since the pre-industrial era, confirming the ocean's absorption of anthropogenic CO₂ and its chemical consequences.
- Reconstructing Past Extreme Events: Damage layers, growth hiatuses, or specific geochemical signatures within coral skeletons can sometimes correspond to historical hurricane passages, major flood events (via runoff signals), or past bleaching events.
A Fragile Archive Under Threat: Climate Change Impacts on Corals Themselves
Photo by Torsten Dederichs on Unsplash
Ironically, the very climate change that corals so effectively record is also posing an existential threat to their survival, jeopardizing these invaluable natural archives:
- Coral Bleaching (Thermal Stress): When water temperatures exceed corals' tolerance threshold for extended periods (often just 1-2°C above the normal summer maximum), the crucial symbiosis with zooxanthellae breaks down. The coral expels the algae, losing its color (appearing bleached white) and primary energy source. Prolonged bleaching leads to coral death. Mass bleaching events, driven by marine heatwaves, are becoming more frequent and severe globally. Tools like NOAA's Degree Heating Weeks (DHW) metric track accumulated heat stress. (NOAA Coral Reef Watch)
- Ocean Acidification (Skeletal Weakness): As oceans absorb more CO₂, the resulting lower pH reduces the availability of carbonate ions (CO₃²⁻) needed for corals to build their aragonite skeletons. This slows coral growth rates, makes skeletons less dense and more brittle, hinders recovery from damage, and makes it harder for coral larvae to settle and build new reefs.
- Increased Storm Intensity: Climate change is predicted to increase the intensity of tropical cyclones. Stronger storms cause greater physical destruction to reef structures, breaking coral colonies and reducing complex habitats.
- Sea-Level Rise: While corals can grow vertically to keep pace with slow sea-level rise, rapid rise can potentially "drown" reefs by reducing light availability for their symbiotic algae if growth cannot keep up. Changes in water depth also alter wave energy reaching the reef.
- Disease Outbreaks: Stressed corals (due to heat, pollution, etc.) are often more susceptible to diseases, which are also becoming more prevalent in some regions.
- Synergistic Stressors: These climate impacts interact with local stressors like overfishing (removing algae grazers), pollution (nutrient runoff can fuel algae overgrowth; chemicals can harm corals), and sedimentation (smothering corals), further reducing reef resilience.
Protecting the Archives: Conservation Imperatives
Safeguarding coral reefs is essential both for their intrinsic ecological value and their role as climate recorders. Strategies include:
- Global Climate Change Mitigation (Paramount): Aggressively reducing global greenhouse gas emissions is the *only* long-term solution to alleviate the primary threats of ocean warming and acidification. Without this, local conservation efforts will ultimately be insufficient.
- Marine Protected Areas (MPAs): Establishing well-managed MPAs can reduce local stressors like overfishing and habitat destruction, allowing reefs to be more resilient to climate impacts and potentially facilitating recovery after disturbances.
- Managing Local Stressors: Reducing land-based pollution (nutrients, sediments, chemicals) through improved watershed management, wastewater treatment, and sustainable agriculture. Implementing sustainable fishing practices.
- Active Restoration Efforts: Techniques like coral gardening (growing fragments in nurseries and outplanting them), microfragmentation (breaking corals into tiny pieces to accelerate growth), and exploring assisted evolution (identifying and propagating more heat-tolerant coral genotypes) are being developed and deployed. However, these are often localized, costly, and cannot succeed without addressing the root causes of reef decline (warming and acidification).
- Continued Research and Monitoring: Ongoing scientific research to understand coral resilience, improve restoration techniques, and continue extracting valuable climate data from existing reef structures is vital.
Conclusion: Heeding the Warnings Written in Stone
Coral reefs are far more than beautiful underwater ecosystems; they are living historical documents, meticulously recording Earth's climate history within their skeletons. The geochemical signals locked within coral archives provide high-resolution insights into past ocean temperatures, salinity changes, pH levels, and extreme events, offering invaluable context for understanding current anthropogenic climate change. Yet, these vital recorders are critically endangered by the very changes they document. Protecting coral reefs through global climate action and targeted local conservation is not only essential for preserving marine biodiversity but also for safeguarding these irreplaceable natural archives that hold crucial lessons for navigating our planet's climatic future.