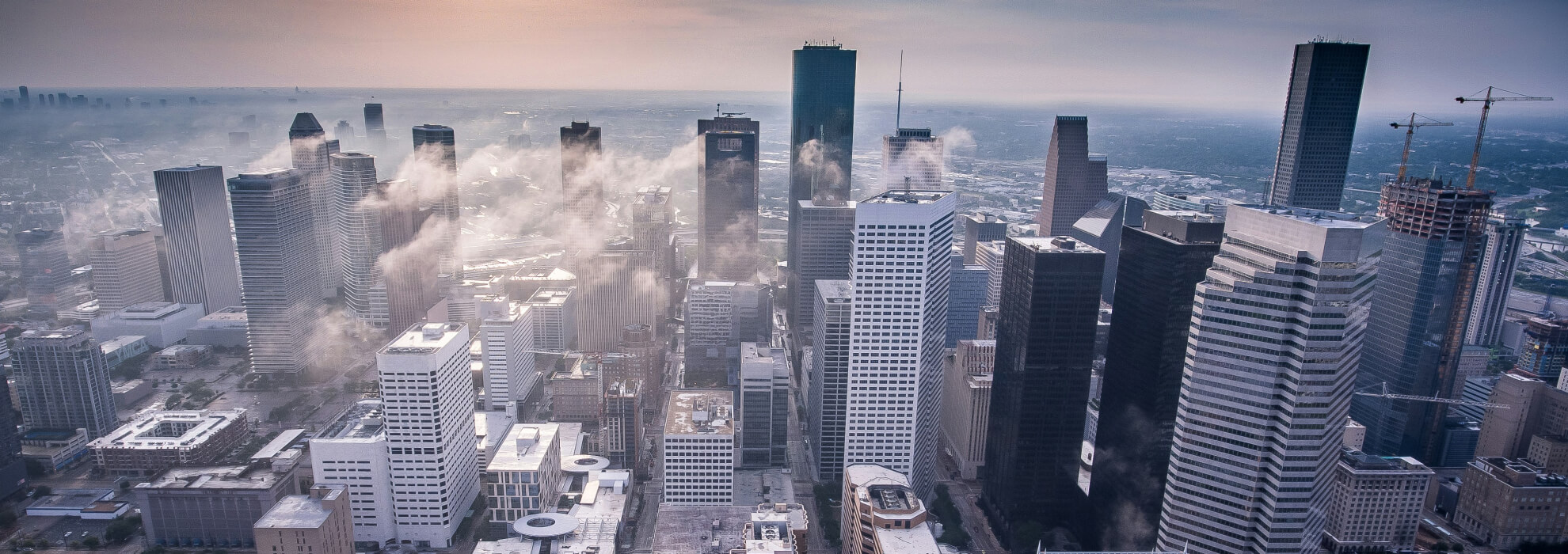
Watch short for this article (5 slides)
The Hidden Climate Threat: Unpacking and Mitigating Methane Emissions from Landfills
Landfills, the final resting places for much of society's refuse, represent more than just buried trash; they are significant and often underestimated sources of potent greenhouse gases, particularly methane (CHâ‚„). As organic waste decomposes under oxygen-deprived conditions, it generates methane, a gas with a powerful short-term impact on global warming, far exceeding that of carbon dioxide (COâ‚‚). Recent attention on methane's role in climate change has intensified scrutiny on landfills and spurred innovation in capturing, reducing, and preventing these harmful emissions. Understanding the science behind landfill methane generation and the strategies available to mitigate it is crucial for tackling the climate crisis.
The Science of Landfill Gas: How Buried Waste Generates Methane
When organic materials – such as food scraps, yard trimmings, paper, wood, and textiles – are buried in a landfill, they undergo **anaerobic decomposition** due to the lack of oxygen deep within the waste mass. This complex biological process occurs in several stages driven by different communities of microorganisms:
- Aerobic Decomposition (Initial Phase): Immediately after disposal, some oxygen is present, allowing aerobic bacteria to break down organic matter, producing mainly COâ‚‚ and heat. This phase is relatively short-lived as oxygen is quickly consumed.
- Hydrolysis & Acidogenesis (Oxygen Depletion): As oxygen runs out, anaerobic bacteria take over. Complex organic molecules (carbohydrates, proteins, fats) are broken down (hydrolyzed) into simpler soluble compounds like sugars and amino acids. Acidogenic bacteria then ferment these compounds into volatile fatty acids (VFAs), alcohols, ammonia, COâ‚‚, and hydrogen (Hâ‚‚). This stage causes the leachate (liquid draining from the landfill) to become acidic.
- Acetogenesis: Acetogenic bacteria convert the VFAs and alcohols produced in the previous stage into acetic acid, COâ‚‚, and more hydrogen.
- Methanogenesis (Methane Production): This is the final and slowest stage. Methanogenic archaea (single-celled organisms distinct from bacteria) utilize the acetic acid and hydrogen produced by the earlier stages. They convert acetic acid into CHâ‚„ and COâ‚‚, and combine hydrogen with COâ‚‚ to produce CHâ‚„ and water.
The overall result is **landfill gas (LFG)**, typically composed of roughly 45-60% methane (CHâ‚„) and 40-55% carbon dioxide (COâ‚‚), along with trace amounts of nitrogen, oxygen, water vapor, and various non-methane organic compounds (NMOCs), some of which can be odorous or toxic. The rate and volume of LFG production depend heavily on factors like waste composition (especially the amount of readily biodegradable organic matter), moisture content, temperature, landfill age, and management practices.
Methane vs. Carbon Dioxide: Understanding the Potency Problem
While COâ‚‚ persists in the atmosphere for centuries, methane is significantly more potent at trapping heat in the short term. This is quantified using the **Global Warming Potential (GWP)**, which compares the heat-trapping ability of a gas to that of COâ‚‚ over a specific time horizon:
- Over a **100-year period (GWP100)**, methane is estimated to be **27-30 times** more potent than COâ‚‚.
- Over a **20-year period (GWP20)**, methane is **~80-83 times** more potent than COâ‚‚.
(Values based on the IPCC Sixth Assessment Report, including climate-carbon feedbacks).
Methane's higher short-term potency is due to its molecular structure, which is highly effective at absorbing infrared radiation, and the fact that it absorbs radiation in different wavelengths than COâ‚‚, essentially closing an atmospheric window that would otherwise allow heat to escape. Although methane breaks down faster (average atmospheric lifetime of ~12 years vs. centuries for COâ‚‚), its intense warming effect during that time makes reducing methane emissions a critical strategy for slowing the *rate* of near-term global warming.
Landfills are a major global source of anthropogenic methane, estimated by the U.S. Environmental Protection Agency (EPA) and the International Energy Agency (IEA) to account for roughly 11-20% of total human-caused methane emissions globally, depending on estimation methods and year.
Strategies for Mitigation: Turning Waste Liabilities into Assets
Recognizing the impact of landfill methane, various strategies are employed globally, often guided by the principles of the **waste hierarchy**: Prevent > Reduce > Reuse > Recycle > Recover > Dispose.
1. Source Reduction and Organic Waste Diversion (Prevention)
The most effective way to reduce landfill methane is to prevent organic waste from entering landfills in the first place.
- Source Reduction: Preventing waste generation initially (e.g., reducing food waste through better planning and storage).
- Composting: Collecting food scraps and yard waste separately and transforming them into valuable compost through controlled aerobic decomposition. Methods include:
- Windrow Composting: Elongated piles turned periodically.
- Aerated Static Pile Composting: Piles with forced aeration, reducing the need for turning.
- In-Vessel Composting: Enclosed systems offering greater process control.
- Benefits: Creates a soil amendment that improves soil health, water retention, and sequesters carbon; avoids anaerobic landfill conditions.
- Anaerobic Digestion (AD): Processing organic waste in enclosed, oxygen-free digesters. Microbes break down the material, producing:
- Biogas: A mixture rich in methane (~50-70%) and COâ‚‚, which is captured and can be combusted to generate heat/electricity or upgraded to Renewable Natural Gas (RNG) for injection into pipelines or use as vehicle fuel.
- Digestate: A nutrient-rich residual material that can be used as fertilizer or soil conditioner.
- Benefits: Captures energy value from waste; produces valuable soil products; occurs in controlled environments.
2. Landfill Gas (LFG) Capture and Utilization (Control)
For landfills already containing organic waste, capturing the generated LFG is crucial.
- System Design: Typically involves drilling vertical wells or installing horizontal trenches into the waste mass. These are connected via a network of pipes under vacuum to draw the LFG to a central point.
- Treatment/Utilization:
- Flaring: Collected LFG is burned in a controlled flare. This converts the potent methane into COâ‚‚ (less potent GWP) and water, reducing the immediate climate impact significantly. Flaring is essential when gas quality or quantity is insufficient for energy recovery but still requires destruction.
- Energy Recovery: If volumes and quality are sufficient, LFG can be treated (removing moisture, particulates, and sometimes siloxanes or sulfur compounds) and used as fuel:
- To generate electricity using engines, turbines, or fuel cells.
- For direct use in boilers, kilns, or industrial processes nearby.
- Upgraded to Renewable Natural Gas (RNG) or biomethane by removing COâ‚‚ and impurities, making it interchangeable with conventional natural gas.
- Efficiency and Challenges: While highly effective, LFG capture systems are not 100% efficient. Fugitive emissions can escape through leaks in the cover system or areas not yet under collection. Capture efficiency typically ranges from 60-85% in well-managed systems but can be lower. Gas production also declines as the landfill ages.
3. Enhancing Decomposition and Oxidation (Advanced Landfill Management)
Newer landfill designs aim to manage LFG production or promote methane oxidation.
- Bioreactor Landfills: Actively manage moisture content (e.g., leachate recirculation) to accelerate waste decomposition and LFG generation within a shorter timeframe, allowing for more efficient gas capture during the landfill's active life. Some designs incorporate air injection (aerobic bioreactors) to minimize methane production altogether, although this is less common.
- Biocovers / Methane Oxidation Layers: Designing final landfill covers using specific soil materials (like compost) that encourage the growth of methanotrophic bacteria. These microbes naturally consume methane, converting it to COâ‚‚ as LFG passively migrates through the cover, thus reducing final atmospheric emissions.
4. Waste-to-Energy (WTE) via Incineration (Alternative Disposal)
WTE facilities combust municipal solid waste at high temperatures, significantly reducing waste volume (by ~90%) and generating heat used to produce electricity.
- Methane Context: By destroying organic waste through combustion, WTE avoids the anaerobic decomposition process that generates methane in landfills.
- Considerations: While avoiding landfill methane, WTE produces COâ‚‚ emissions (from both fossil-based materials like plastics and biogenic sources) and requires sophisticated air pollution control systems to manage emissions of pollutants like dioxins, furans, heavy metals, and particulate matter. Its position in the waste hierarchy is generally above landfilling but below prevention, reuse, recycling, and organic diversion.
Policy and Regulation: Driving Change in Waste Management
Government policies are critical drivers for reducing landfill methane:
- Landfill Regulations: Rules mandating LFG collection and control systems for larger landfills (e.g., EPA's New Source Performance Standards (NSPS) and Emission Guidelines (EG) in the US).
- Organic Waste Diversion Mandates: Regulations requiring municipalities or businesses to separate organic waste for composting or anaerobic digestion (e.g., California's SB 1383, EU Landfill Directive targets).
- Incentives: Financial mechanisms like carbon credits, renewable energy credits (RECs) for LFG energy projects, feed-in tariffs for biogas electricity, and grants for composting/AD infrastructure encourage investment.
- Extended Producer Responsibility (EPR): Policies making manufacturers responsible for the end-of-life management of their products, encouraging design for recyclability and waste reduction.
- Circular Economy Frameworks: Broader policy shifts promoting resource efficiency, waste minimization, reuse, and recycling over disposal.
Global Perspectives: Comparing Approaches
Countries employ diverse strategies based on resources, population density, and policy priorities:
Country/Region | Primary Waste Management Focus | Key Methane Reduction Strategies | General Outcome/Status |
---|---|---|---|
United States | Landfilling common, but increasing diversion/LFG capture. | Mandated LFG capture at large sites; growing state/local composting & AD initiatives; voluntary RNG market. | Moderate progress; significant LFG capture (~60-70% from regulated sites), but large amounts of organics still landfilled. |
Germany | High recycling rates; landfilling of untreated waste largely banned. | Strict organic diversion via mandatory separate collection; WTE for residual waste; mechanical-biological treatment (MBT). | High success; dramatically reduced methane from landfills due to minimal organic input. |
Sweden | Very high recycling/recovery rates; minimal landfilling. | Extensive WTE network; promotion of biogas from AD; landfill ban on organic/combustible waste. | Very high success; extremely low landfill rates (<1%) minimize methane potential. |
India | Landfilling (often open dumping) prevalent; infrastructure developing. | Focus shifting towards source segregation, composting, biomethanation (AD), and some LFG-to-energy projects; significant challenges remain. | Low/Developing; waste segregation and infrastructure are major hurdles; large potential for methane reduction remains. |
European Union (Overall) | Moving up the waste hierarchy via Landfill Directive. | Binding targets for reducing landfilled biodegradable waste; promotion of recycling, composting, AD, and WTE. | Variable success by member state, but overall trend towards reduced landfilling of organics and increased LFG capture/utilization. |
The Future Landscape: Innovation in Landfill Methane Management
Ongoing innovation aims to improve methane mitigation:
- Advanced Monitoring: Using remote sensing technologies (satellites, aircraft, drones equipped with sensors) to detect methane plumes and pinpoint leaks from landfills and LFG infrastructure more effectively and frequently.
- AI and Data Analytics: Employing artificial intelligence to optimize landfill operations, predict LFG generation patterns, improve waste sorting efficiency, and manage LFG collection systems dynamically.
- Enhanced Methane Oxidation Systems: Developing more efficient biocover designs and potentially engineered systems to maximize microbial methane consumption.
- Circular Economy Integration: Deepening efforts to design products for durability, reuse, and recyclability, fundamentally reducing the volume and organic content of waste destined for disposal.
Conclusion: A Critical Front in the Climate Battle
Reducing methane emissions from landfills is one of the most cost-effective and readily achievable actions we can take to slow near-term global warming. It requires a comprehensive strategy that prioritizes preventing organic waste generation and diverting it from landfills through composting and anaerobic digestion. For existing landfills, capturing and utilizing or flaring landfill gas is essential. Advanced landfill management techniques and supportive policies further enhance mitigation efforts. While technological innovation offers promising tools, achieving significant reductions demands collective action from governments implementing strong regulations and incentives, industries adopting sustainable practices, and individuals participating actively in waste reduction and recycling programs. Addressing landfill methane is not just waste management; it is critical climate action.